Sequel of the preceding post Cosmogenesis (9) : The Big Bang Discovery and End of the Cosmogenesis Series.
According to modern physics the universe has undergone a gradual process of expansion and cooling ever since the big bang; at the same time increasingly complex physical structures have evolved. The history of the universe can conveniently be divided into two main periods: the first million years (infancy) and the remaining 15 billion years (maturity).
The Infant Universe
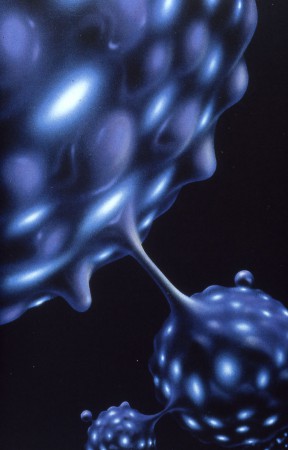
Artistic view by S. Numazawa.
During the Planck era, time and the dimensions of space as we know them were so intimately linked as to be practically indistinguishable. Various speculative theories of “quantum cosmogenesis”, as yet in their infancy, attempt to explain how our universe emerged at the end of the Planck era. Some physicists refer to its “spontaneous emergence”, others to an infinite number of separate “cosmic bubbles” arising from the quantum vacuum like foam from the surface of the sea.
Between 10-43 and 10-32 seconds after the big bang the infant universe consisted of elementary particles bound by a primeval superforce. A few billiseconds later gravity separated itself from the surviving electrostrong force, which in turn, as the temperature fell to 1027 degrees, divided into the strong force and the electroweak force. Recent experiments in high energy physics suggest that these “symmetry breakdowns” had spectacular consequences: the appearance of strange objects; “topological defects” such as “cosmic strings”; even the onset of “inflation” – a very short period during which the universe grew immeasurably. The fundamental constituents of matter – quarks, electrons and neutrinos – also appeared at this time.
10-11 seconds after the big bang the temperature of the universe had dropped to 1015 degrees and the electroweak force split into an electromagnetic and a weak force, thus establishing the four fundamental forces and fixing the physical conditions for the formation of complex structures.
10-6 seconds after the big bang all quarks were “linked” in threes by the strong force to form the first nucleons, i.e. protons and neutrons. By this time the temperature had fallen to a billion degrees as the universe continued to expand. As particles became more widely spaced, they collided less frequently but one hundred seconds or so later the crucial process of nucleosynthesis began. Neutrons and protons combined to form the simplest atomic nuclei: hydrogen, helium and lithium (in various isotopes). Most of the universe, however, remained as isolated protons, i.e. as hydrogen nuclei.
Nucleosynthesis took place only for a very short time: the universe was cooling so rapidly that there was only time for the lightest elements to form. These therefore constitute 99 per cent of the visible matter in the universe today (75% hydrogen and 24% helium). The remaining one per cent, consisting of heavier elements like carbon, nitrogen and oxygen, would not be created until billions of years later, when the stars were formed.
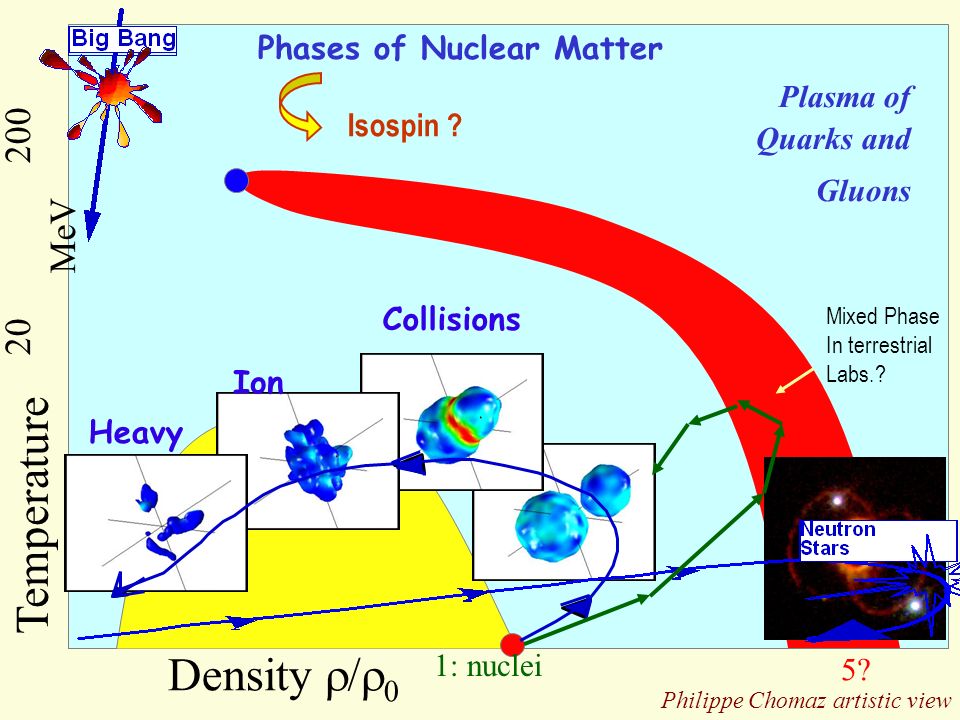
Montage by Philippe Chomaz (GANIL)
Until it was 300,000 years old the universe remained opaque; in other words it emitted no radiation: the density of electrons prevented photons from moving freely. But the universe, consisting of a “soup” of particles and radiation, continued to cool and expand until, at 3,000 degrees, it became transparent and emitted its first electromagnetic signal in the form of what we now detect as cosmic background radiation.
A million years after the big bang the first atoms were formed, when electrons were captured by hydrogen and helium nuclei, and these atoms combined into molecules to create vast clouds of hydrogen, out of which stars would later emerge.
The Birth of Stars
The universe today is no longer homogeneous like a sea of oil, as it was 15 billion years ago. Cosmic evolution has created all sorts of individual structures. Each single star probably has its own planetary system, like our own solar system. Hundreds of billions of these are combined into galaxies, like the Milky Way. In the part of the universe we can see it is estimated that there are a thousand billion galaxies. Some are elliptical, spiral (including ours) or lenticular; others, whose origin is still a mystery, are irregular in shape. Galaxies are grouped into clusters and superclusters to form the fabric of the universe: astronomers have identified several thousand clusters, each of which comprises hundreds or even thousands of galaxies; these clusters in turn form part of even larger structures, superclusters. Superclusters are arranged as if on the surface of gigantic cosmic bubbles, hundreds of billions of light-years across, which appear to be almost “empty” of matter (see p. 122), making the universe as a whole resemble a sponge. One of the biggest challenges facing today’s cosmologists and astrophysicists is to understand how these bubbles were formed.
Different Models of Galaxy Formation. The formation of cosmic structures, especially galaxies and clusters of galaxies, was a problem that preoccupied cosmologists for more than half a century. Not knowing the exact process of evolution, they simulated various possibilities using powerful computers. Eventually they succeeded in creating a process which led to structures resembling those of the galaxies and clusters in the observable universe. Now cosmologists are trying to understand how even larger structures, such as filaments and bubbles, might have developed from minute fluctuations in the original cosmic brew. These pictures represent three different hypotheses as to the nature of the “missing mass” or “dark matter” of the universe: “cold dark matter” (a), “hot dark matter” (b) and a mixture of the two (c) – the last being the model which best accounts for the observed pattern of galaxies. Computer simulation by J.-M. Alimi, Paris Observatory.
One of the keys to this understanding is the decoupling of matter and radiation, which took place during the period when cosmic background radiation began. This was in fact when cosmic structures began to take shape, the moment when the force of gravity started to exaggerate the minute variations in density of the primordial gas: every “lump” in the soup would attract nearby matter, which would make it even more dense. After a few million or billion years the lump would have developed a recognizable structure.
When these lumps of matter reached a certain density, their own gravitation would counteract the overall expansion of the universe and make them contract even more. It is not known, however, how large or massive these early structures would have been. Were there originally individual stars, which grouped themselves into galaxies and then into clusters and superclusters? Or were there vast clouds which disintegrated into galaxies and then into individual stars?
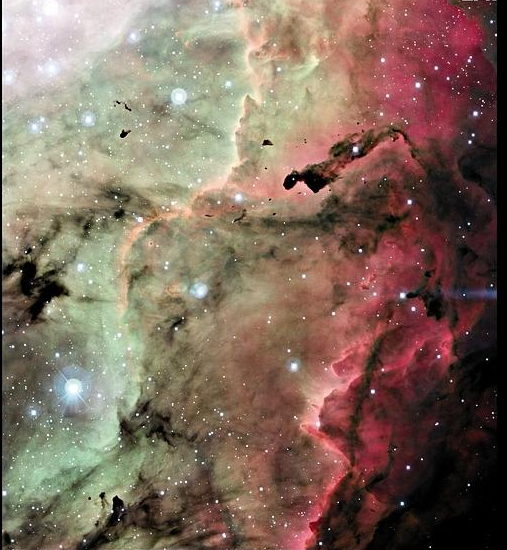
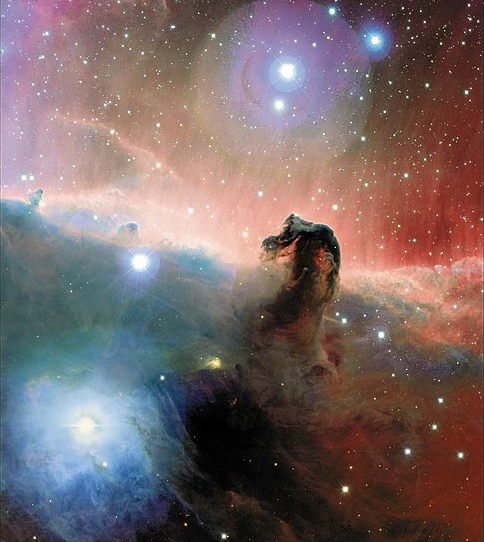
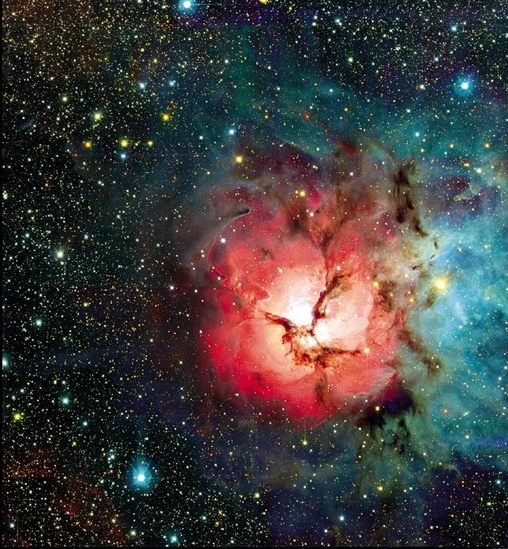
As inhabitants of the Solar System, we are still more interested in the formation of the stars and the planets that might be orbiting them. The material from which they were formed is gas – the same gas that fills the Milky Way and every other galaxy. This gas exists in clouds of varying density, temperature and chemical composition. Some of them, illuminated or excited by neighboring stars, appear as gaseous nebulae. It is here that stars are formed.
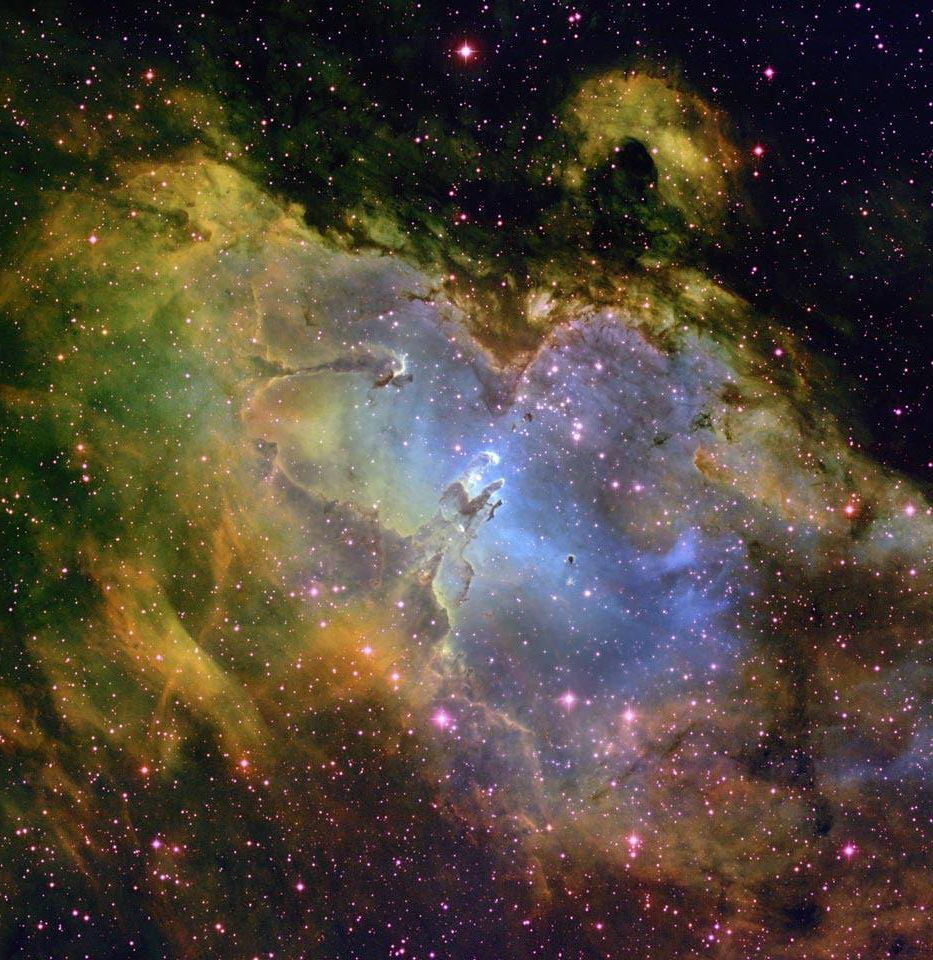
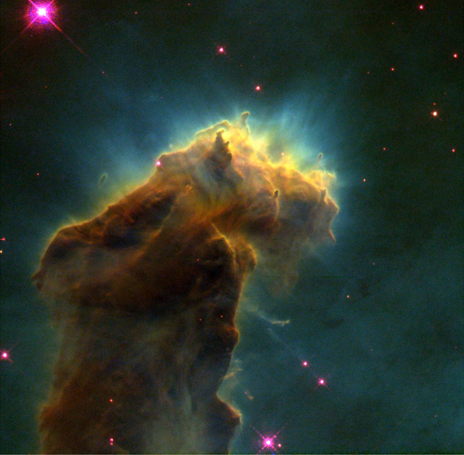
Gravity is the driving force behind the formation of stars: under the influence of gravity nebulae rapidly condense and collapse, eventually forming stars, which initially consist mainly of hydrogen. As it condenses, the gas in the nebula gets hotter; when the center of the cloud reaches 15 million degrees, a chain of nuclear reactions is triggered which effectively marks the birth of the star: it is the fusion of hydrogen to helium that generates the energy which makes the star shine. That energy also stabilizes the star by counteracting the force of gravity and preventing further contraction. This is the beginning of the star’s maturity, known as its “main sequence”, whose duration depends on the star’s mass: ten billion years for a smallish star like the sun; much less for larger stars.
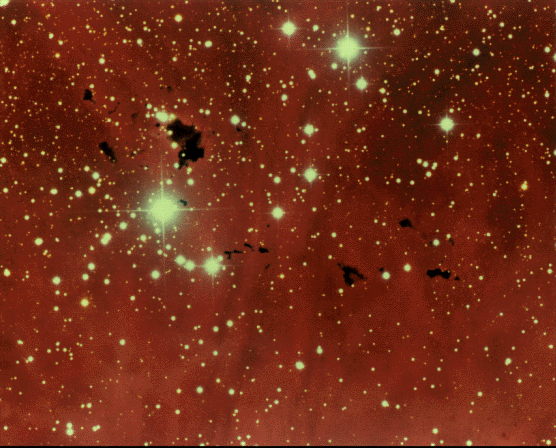
Bok globule in IC 2944, © D. Malin
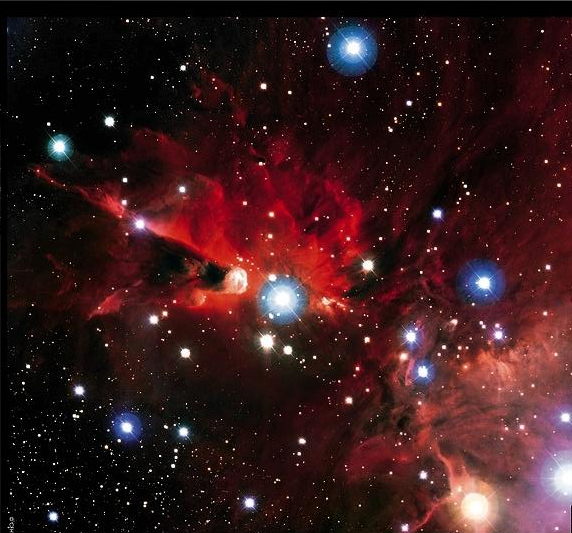
Star cluster NGC 2264 in the Conus Nebula. © D. Malin
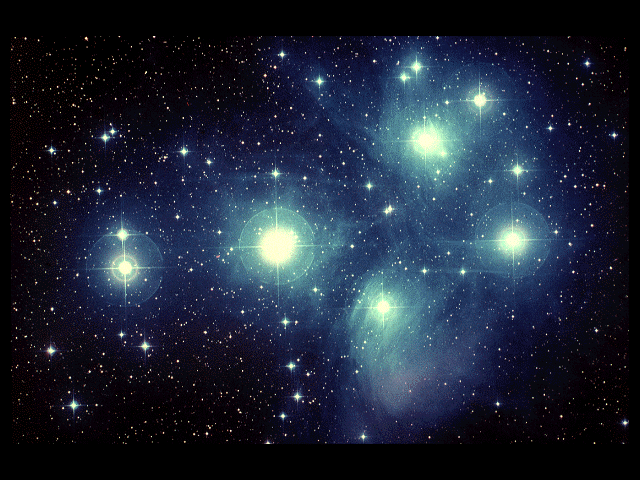
Open cluster of the Pleiades, © D. Malin
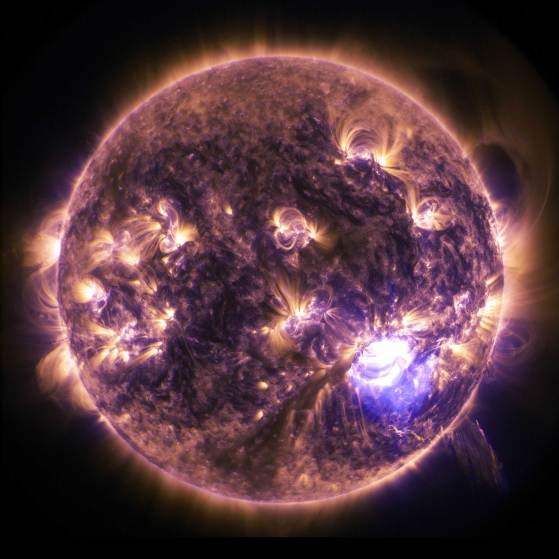
At the end of the main sequence the helium starts to burn, producing carbon, oxygen, magnesium, etc. This is where the history of chemical elements begins, a chain of nuclear reactions realizing the alchemist’s dream of creating heavier and heavier (and increasingly rare) elements from the simplest material, hydrogen. Inside the largest stars all the elements up to and including iron are created, but no further thermonuclear reaction can take place. From then on no more energy can be generated and the massive gravitational pull of the star no longer counteracted: its iron core collapses under its own weight to form an ultra-dense nucleus, a neutron star or, in extreme cases, a black hole. As the star implodes, its crust rebounds off the nucleus in a massive explosion: the star has turned into a supernova.
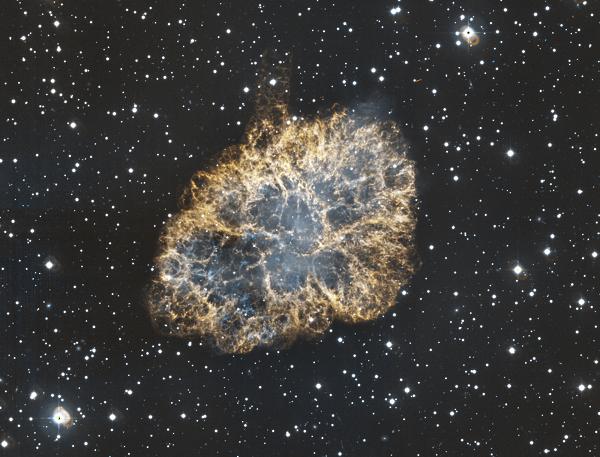
© J. Hester and P. Scowen (Arizona State University) and NASA.
This cataclysm, which occurs only to the most massive stars (at least ten times the mass of the sun), hurls into space all the heavy elements that were formed in the now defunct star and these provide raw material for the formation of a new generation of stars.
The very first generation of stars did not form until two or three billion years after the big bang; the largest of them grew rapidly until they exploded as supernovae, creating new clouds of interstellar gas. The second generation was formed largely out of hydrogen and helium but also incorporated some of the heavier elements created inside first generation stars. The pattern of evolution continued with subsequent generations, enriching the chemical composition of the universe and of the objects it comprised with increasing quantities of heavy elements.
Our sun emerged about 4.5 billion years ago from a cloud of gas known as a “giant molecular cloud”. It may have been powerful shock waves from a nearby supernova explosion that triggered the sun’s formation. The planets were formed, probably at the same time as the sun, out of gas and dust in the nebula. In fact every object in our solar system — the small rocky planets, the large gaseous planets, the asteroids and comets, etc. – emerged simultaneously from the same cradle: a gigantic cloud of hydrogen.
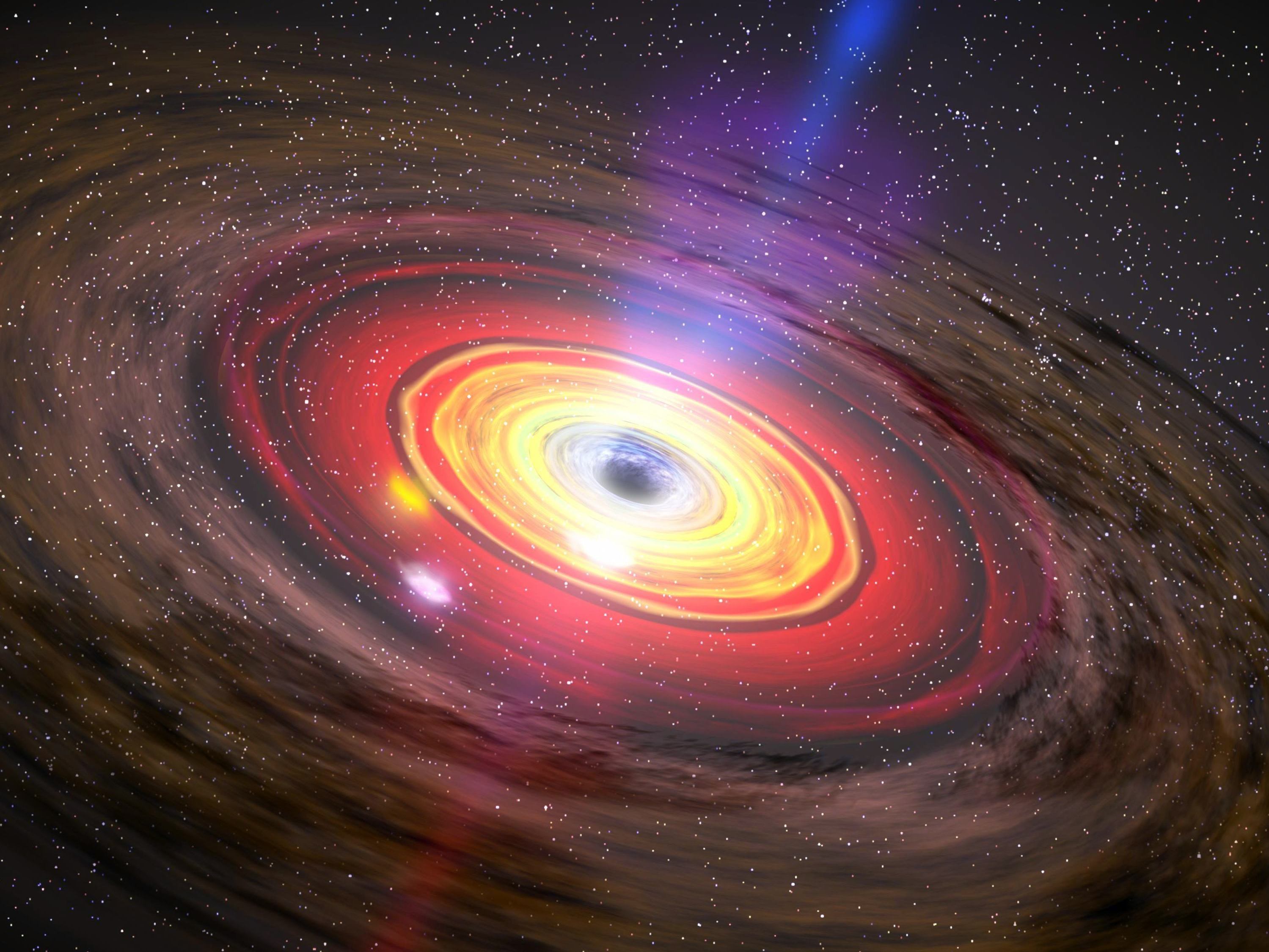
© Dana Berry / STScI / NASA
During the first 500 million years of its existence the solar system consisted of a flat disc of gas, rotating about its own axis, in which pockets of gas and lumps of rock were flying in all directions. These collided with one another and gradually coalesced into larger and larger objects, culminating in the formation of the planets.
Slowly each planet developed its own identity. The sun’s heat burnt off the lighter, gaseous elements surrounding the inner planets (Mercury, Venus, Earth and Mars), which turned into balls of molten rock; a process of fusion forced the heavier elements (iron, nickel, etc.) to the center of the planets, leaving most of the lighter elements like silicon and carbon near the surface. But the outer planets remained entirely gaseous and even today consist largely of lighter gases like hydrogen and helium.
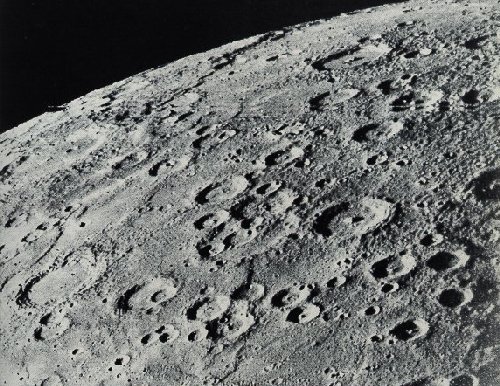
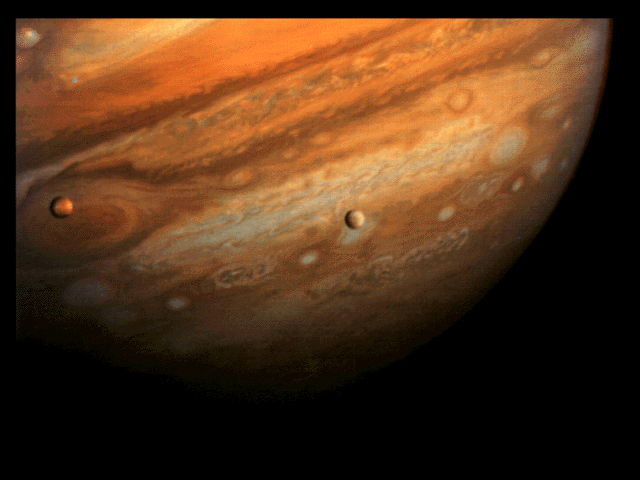
A number of small objects avoided being amalgamated into any of the planets, which had no sooner formed than were bombarded by a veritable deluge of meteorites, asteroids, planetoids and comets. Hordes of these “minor planets” whirled about all parts of the solar system and the surfaces of Mercury, Mars and the moon still bear the scars from impacts they sustained during this period. The earth too suffered a similar bombardment before being protected by its atmosphere, which appeared much later.
The moon itself was probably formed when a planetoid the size of Mars collided with the earth soon after it had formed, tearing part of it away and disintegrating in the process. The lump of molten rock which had been detached began orbiting the earth and coalesced to form the moon.
Today the solar system is much “tidier”: the few remaining minor planets have gathered either in one of the asteroid belts or in distant regions beyond Pluto, now and then visiting us as comets but only occasionally threatening to collide with the earth.
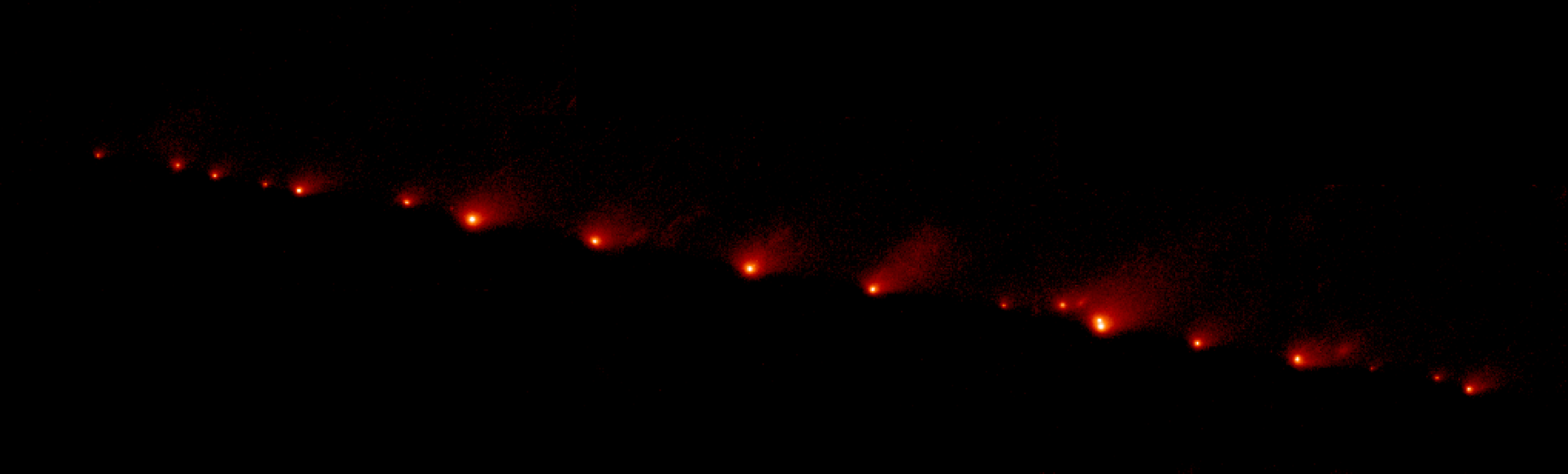
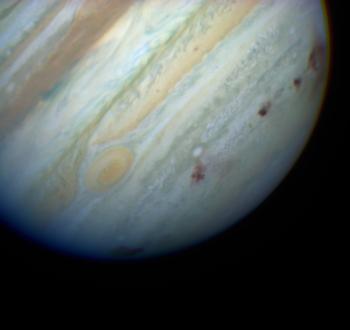
Comet Shoemaker-Levy 9 colliding with Jupiter, ©
STScI / ESA
Some three tons of rock fragments were collected from the meteorite which landed in Allende in Mexico on 8th February 1969. This was the first time anyone had witnessed material dating from before the formation of the solar system; it had probably been formed when the shock wave from an exploding supernova triggered the condensation of the original nebula. In this tiny fragment weighing just 300g (fig. 272) both chondrules and white granules are clearly visible, the latter being 4.566 billion years old – the same age as the solar system. The magnesium content of some of these granules is rich in isotope 26Mg3 a product of the disintegration of the radioactive isotope 26A1 of aluminium, which is produced by supernovae and ejected into space when they explode. It is believed that the first solar particles were created less than five million years after the explosion of a nearby supernova. In July 1994 the comet known as Shoemaker-Levy 9 collided with Jupiter, an event which was recorded at the Hawaii observatory using an infrared sensor and a telescope equipped with “adaptative optics” (to neutralize the effect of the earth’s atmosphere). First the comet’s nucleus was broken into 21 pieces by the tidal forces generated by the giant planet (fig. 274). Then the fragments plunged into Jupiter’s atmosphere, causing huge explosions -some of them equivalent to the detonation of 100 million megatons of TNT: observers reported great mushroom clouds appearing about ten seconds after each impact (fig. 275). Scientists believe that the earth suffered similar collisions four billion years ago and, since comets contain large amounts of water, carbon and organic molecules, some researchers reckon that they could have brought the organic matter from which all terrestrial life forms have evolved.
- Allende meteorite
Paris, National Museum of Natural History, Mineralogy Department
Rocks From the Sky
Chondrites are stony meteorites which are as old as the solar system: about 4.56 billion years. Carbonaceous chondrites are the oldest of all and their chemical composition is similar to that of the sun, minus volatile gases like hydrogen and helium. They consist of chondrules, tiny spheres (between 0.2 mm and 4 mm across), which were probably formed inside the protosolar nebula when grains of dust collided and fused. The white dust granules are among the first solid objects to be formed in the solar system; chemical analysis of these granules has revealed traces of protosolar dust.
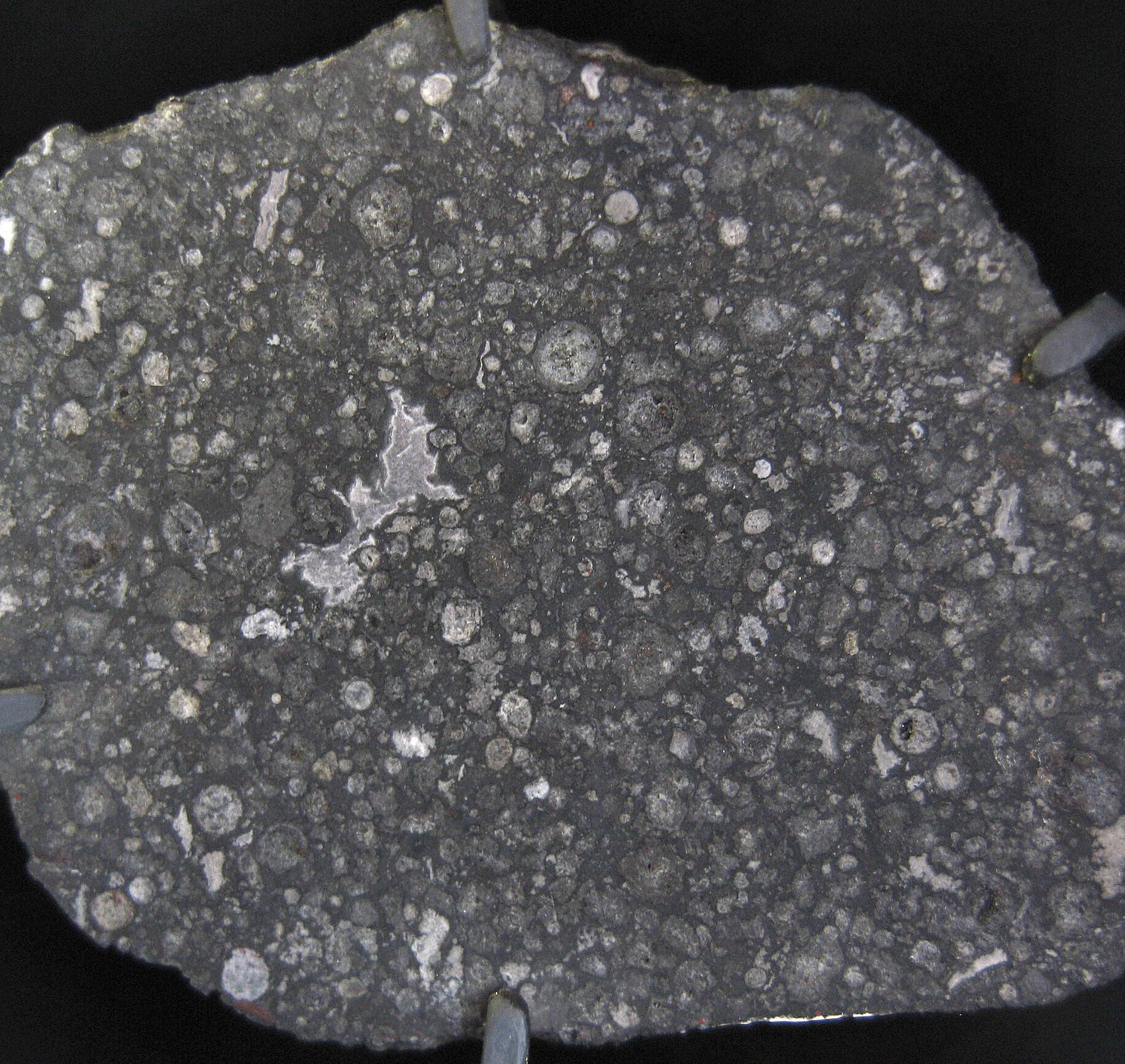
Other types of meteorite originate from asteroids or planets, which evolved independently of the earth and therefore have different chemical compositions. As these objects cooled, two nickel-iron alloys were formed, each with a different geometric structure – a complex pattern of oblique and parallel lines known as Widmanstatten figures. These patterns, which are revealed when a polished slice of the meteorite is attacked by acid, tell us what the interiors of other planets look like – worlds which are otherwise inaccessible to us. As the French poet Roger Caillois has put it, they are “the only drawings known to man which were not made on earth” (“Recipe”, Minerals, Gallimard, 1970).
The Appearance of Life
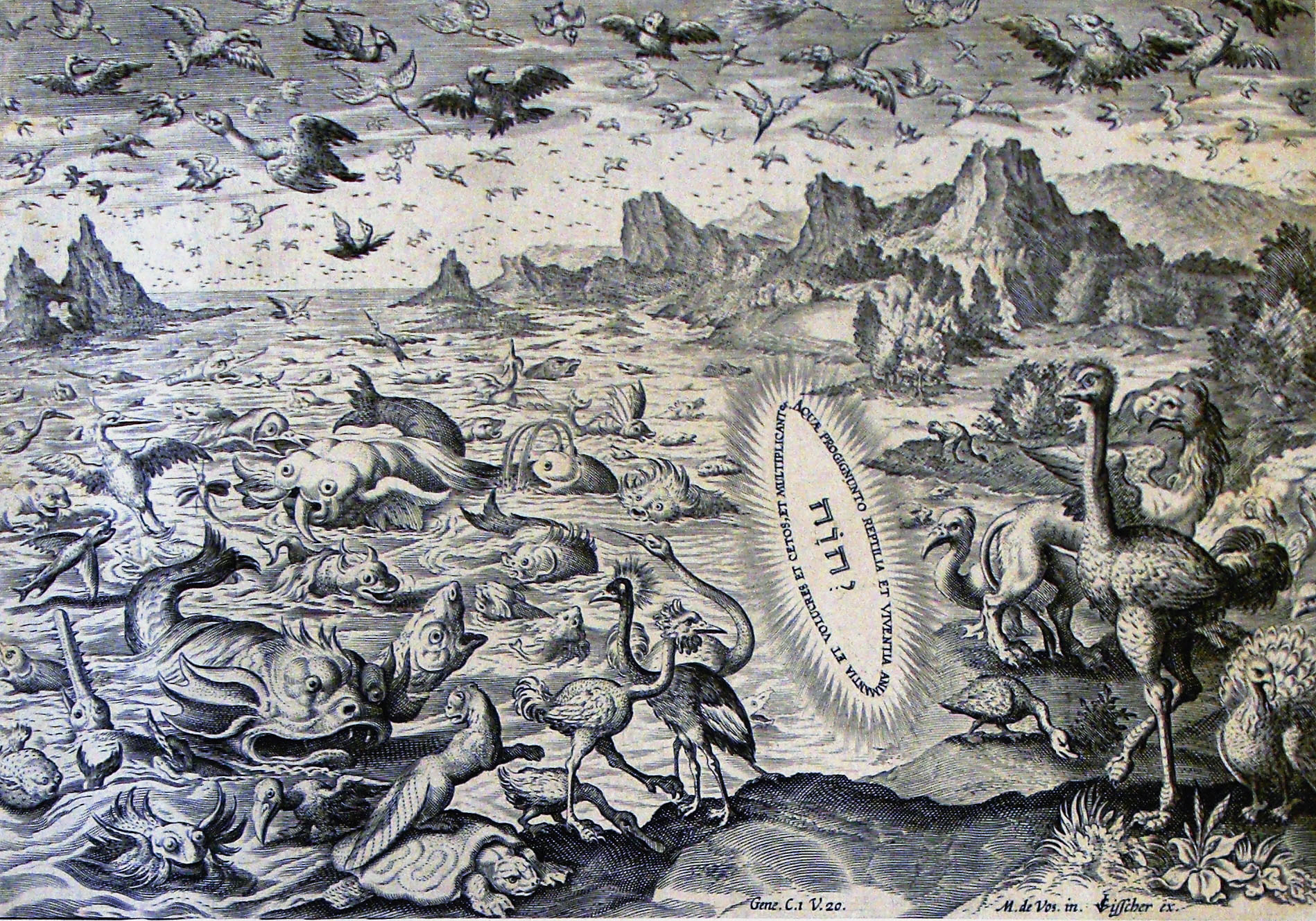
In the mid-18th century the Swede Carl von Linné identified and classified 12 thousand species of living things, many of them obviously related. Then, in 1809, the French naturalist Jean Lamarck published his Philosophie zoologique, which broke with the traditional belief in the Creation story by suggesting that there was a hierarchy of species, from the simplest to the most complex, which had resulted from a gradual process of transformation. The simplest organisms had appeared first (spontaneously) and had progressively metamorphosed as they adapted to their environment and passed on the resulting modifications to their offspring in a process Lamarck called “acquired characteristic inheritance”; species that had failed to adapt to changes in their environment had become extinct, as evidenced by fossils. This hypothesis was contested by Georges Cuvier, French anatomist and father of palaeontology and comparative anatomy, who argued that species had become extinct as a result of the various catastrophes the earth had suffered since its creation.
The publication of Darwin’s The Origin of Species in 1859 provoked violent argument. In it he echoed Lamarck’s idea of gradual transformation but suggested that it worked in a different way: according to natural selection and the survival of the fittest. The development of genetics in the early 20th century led to general acceptance of the theory of evolution, even though its precise workings are still being debated and the significance of catastrophic events (both geological and astronomical) is now fully appreciated. Today we know that all living things, including man, evolved from the same primitive organisms.
Another crucial step was taken in 1865, when Pasteur put an end to two thousand years of controversy by demonstrating that fermentation was caused by organisms rather than resulting from spontaneous generation. With the advance of biological science, vitalism found fewer and fewer adherents as they were forced to accept that living matter and inert matter do not consist of different types of atoms but merely have different arrangements of atoms. At the same time, both chemists and biologists tried to discover how the first organic molecules were formed. In 1953 an experiment conducted by the American chemist Stanley Miller caused great excitement: electrical discharges (similar to miniature thunderstorms) were passed through a mixture of water, methane, ammonia and hydrogen (a recreation of what was then thought to have been early earth’s atmosphere) and, after a week, a few prebiotic compounds were found to have been formed; among them were amino acids, the basic components of proteins. Today it is believed that the earth’s atmosphere initially consisted mainly of carbon dioxide and nitrogen, which are far less volatile than the gases used in laboratory experiments like Miller’s (although this does not necessarily invalidate them, since conditions more favorable to the creation of life forms might have existed in certain places).
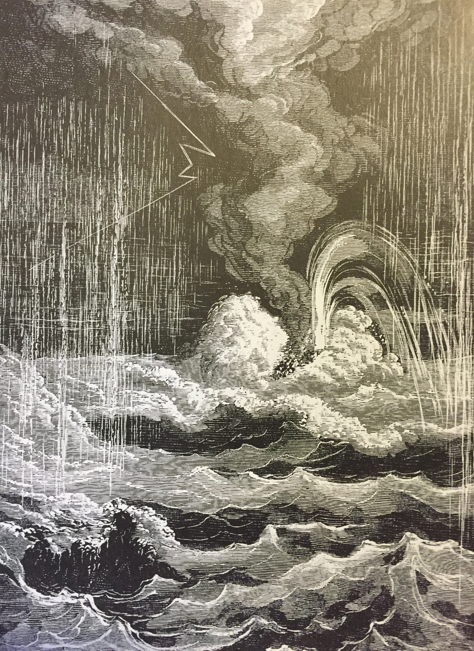
Camille Flammarion, Les Terres du ciel, 1884.
It has recently been suggested that life originated in space. Astrophysicists have discovered organic molecules (amino acids, nucleic acids and fatty acids, etc.) in meteorites and other extraterrestrial objects. When these were bombarding the earth during the first few hundred million years of its life, they could have brought with them some of the organic matter from which the first life forms emerged. Indeed the appearance of the first living organisms coincided with the end of meteorite bombardment, approximately 3.8 billion years ago.
The fact that increasingly complex molecules could have developed from ordinary matter by means of standard chemical processes is not in itself problematical. What is in doubt is how life emerged from inanimate matter – in other words, how a new “order” was created. There are plenty of examples in thermodynamics of order arising out of disorder, but no one yet understands every detail of the appearance of the highly complex DNA molecule, of viruses or of cell tissue. Several scenarios have been proposed, but experimental results do not make it possible to choose between them.
Membranes to separate organisms from their environment, genetic fingerprints, metabolic and reproductive systems: primitive structures like these appeared during an early stage, even before the first living cells had developed, after several generations of prebiotic structures. They were initially anaerobic (the earth’s atmosphere then contained almost no oxygen) and heterotrophic (i.e. they had to extract from their environment the organic matter they needed for growth). Five hundred million years later some of these cells evolved a new mechanism, photosynthesis, which allowed them to escape that need by using the sun’s energy to break down water and carbon dioxide molecules to produce sugar and oxygen. Their success was demonstrated by the rapid proliferation of stromatolites, vast organo-sedimentary structures.
The metabolic activity of these new autotrophic bacteria, which released large quantities of oxygen into the atmosphere, brought about radical changes in the terrestrial environment: many cell types disappeared, “poisoned” by the new gas; some took refuge in the sea or underground; others adapted to it and became aerobic. The formation of a protective ozone layer, on the other hand, encouraged certain life forms, including primitive eucaryotes (cells with a membrane-enclosed nucleus), to venture out of the depths of the ocean. Of the three main branches of the family tree of life, archaebacteria, eubacteriales and eucaryotes, it is the last which is of particular relevance to human evolution. Eucaryotes developed sexual reproduction about a billion years ago, then multi-cellularity: marine plants, marine invertebrates, fish, ferns, insects, amphibians, reptiles, mammals, flowering plants. Just over a hundred million years ago plant and animal life as we know it was in existence – with one significant exception…
Various species of hominids developed in Africa about five million years ago, modern man (Homo Sapiens Sapiens) being the only one to have survived. In the 40,000 years of its existence — a blink in terms of the history of life on earth – our species has overrun virtually the entire planet. Although we share 99% of our genetic code with our cousin the chimpanzee, it seems that the remaining 1% is crucial – so much so that man has always considered himself to be the nec plus ultra of the evolutionary process. But, just as there is no constitutive difference between living and inert matter, so we should not imagine that there is a radical distinction between our large brain and the smaller brains of the other primates.
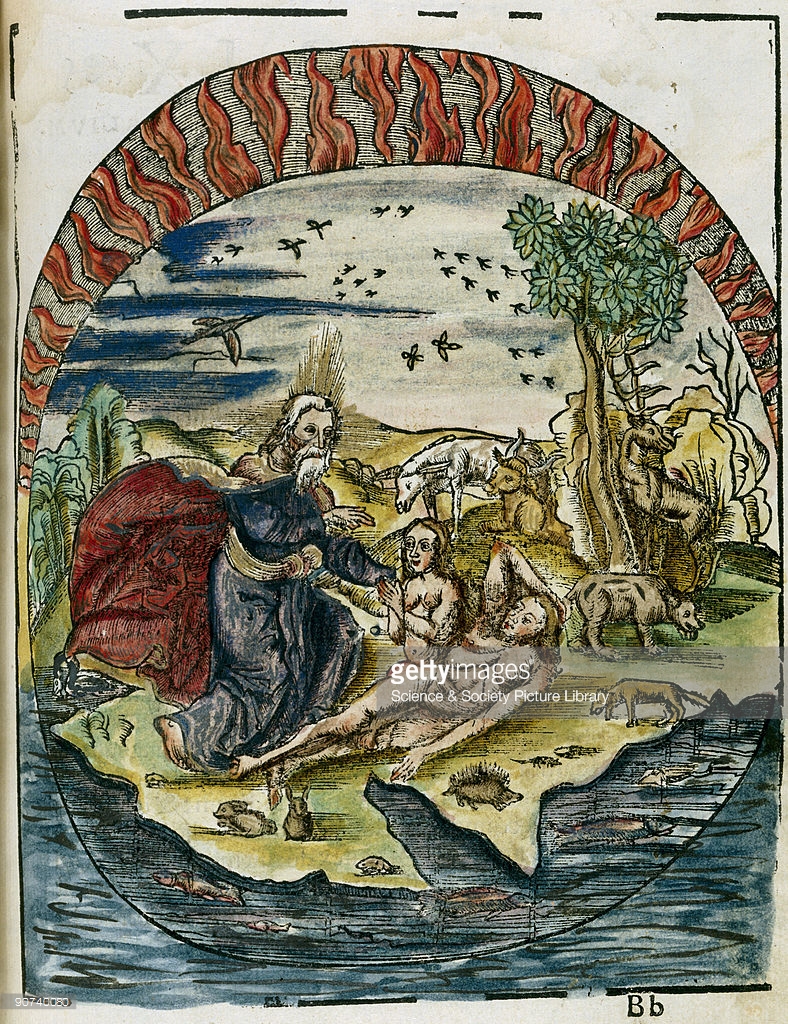
Gregor Reisch, Margarita Philosophica, Freiburg, 1512. Paul Getty Museum.
The emergence of life and the evolution of different species should be seen as part of a larger process whose context is the universe as a whole. The history of man’s search for origins is one of a progressive weakening of the notion “beginning” – beginning of human existence, beginning of life, beginning of time – by substituting evolutionary processes for spontaneous, instantaneous acts of creation. According to modern science, there was no Adam and Eve, no “zero hour”…
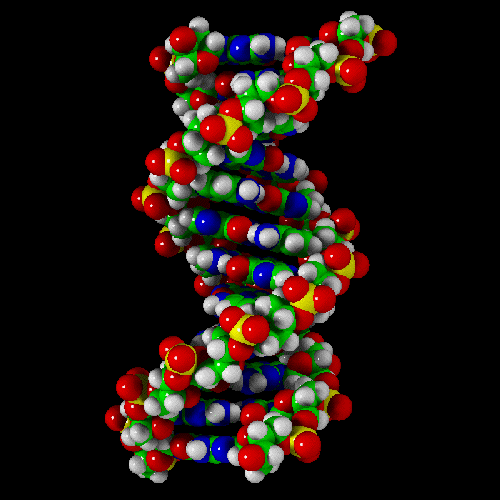
This is an artitic representation of the double helix of the DNA molecule.
The history of life on earth is now regarded not as an inexorable progression towards diversity and complexity but as a largely accidental process of “pruning” whereby only a few branches of an initially luxuriant tree manage to survive. The last 500 million years are now known to have witnessed a series of cataclysms which had a decisive influence on evolution, making it anything but the regular, ordered progression once imagined. During this period the earth has been bombarded by countless comets, meteorites and asteroids, which have caused widespread ecological damage and led to the extinction of numerous species. According to a theory proposed in 1980 by the American physicist Luis Alvarez, it was the impact of a giant meteorite 65 million years ago which caused the destruction of three-quarters of all land and sea animals including the dinosaurs, marking the end of the cretaceous period. This theory has since been corroborated by a number of discoveries, including that of a massive crater in the Gulf of Mexico in 1991-92.
As the American palaeontologist Stephen Jay Gould has written, if it had not been for this catastrophe which wiped the dinosaurs off the face of the earth, “mammals would still be small creatures, confined to the nooks and crannies of a dinosaur’s world. […] We are the glorious accidents of an unpredictable process with no drive to complexity, not the expected results of evolutionary principles that yearn to produce a creature capable of understanding the mode of its own necessary construction.”[1]
The irony is that we now seem to be the only species on earth capable of comprehending the universe from which we have emerged and of asking how it originated. Will we ever find the answer? There is no reason to suppose that we shall. As the French poet Jean Orizet reminds us, “We enquire of the spinning stars how they began, but our question merely glances off them and returns to us unanswered.”[2]
References
[1] Stephen Jay Gould, Life’s Grandeur, Jonathan Cape, London, 1996, p. 216
[2] Jean Orizet, Hommes continuels, Belfond, 1994.